By Vidya Rajan, Columnist, The Times
The Earth rotates once on its axis every day, with the resultant periodicity of night following day. The predictability of exposure to the energy sources of light and heat have allowed lifeforms to evolve mechanisms to harvest it during daytime and develop materials to store that energy. All energy that emanates from the Sun is in the form of electromagnetic waves. Some waves, ex. ultra violet, have the ability to pack so much energy that they can damage DNA and others, ex. infra-red, pack just enough energy to warm our bodies. Between these too-strong/too-weak bookends lies the Goldilocks zone of visible light – the spark of life for Earthlings.
How to take light and parley it into lifeforms?
Enter resonance transfer. This is a process that uses molecules called isomers that share the same chemical formula but have different structures. A familiar isomer pair is glucose and fructose – their shared chemical formula is C6H12O6, but fructose is more than twice as sweet as glucose because its atoms are arranged differently. Other isomers rearrange their structures when exposed to energy but when the energy dissipates, they revert to their original form. This involves the molecule flipping from one form to another, like a stretched rubber band which regains its original shape when released. Using the energy of the stretching of a rubber band, it is a simple matter to launch a spitball at an unsuspecting classmate, an activity that was much enjoyed in the classrooms of my youth. In biological systems, that spitball is an electron which sticks atoms together to make complex molecules such as sugar. Since the atoms of sugar are held together by energy, sugar serves to store energy; in other words, it is a battery.
The way a battery functions is conceptually brilliant and technically simple. Think of your phone charger. When you plug one end of the charger into the wall and the other end into your phone, the battery gets powered up. Power is simply the movement of electrons – yes, that is why it is called electricity. So when you plug the charger into the wall outlet, electrons, which are negatively charged particles, move from one place to another. And positive charges, well, they obviously move in the other direction. So, by extension, if you could force positively charged particles to move, say, from right to left, you’ve effectively moved negatively charged particles in the other direction, left to right. Your phone is powered up with all the positive charges collected on one side of the battery (the “+” pole) and the negative charges collected on the other side (the “–“ pole) and separated by an impermeable structure neither can diffuse across without passing through the phone and lighting it up. Therefore, power stored in a battery is vested in the location of the electron.
Harking back to our conversation about molecular isomers that harvest energy, a simple isomeric molecule is retinal, made only of carbons and hydrogens. In the ancient domain of Bacteria, retinal is found conjugated to proteins in a molecule called bacteriorhodopsin. This molecule is a pump for positively charged particles made of the simplest atom in existence, hydrogen ions (H+). When H+ ions are pumped in one direction then “+” ions gather on one side of an impervious “cell membrane” and electrons or “–“ ions gather on the other; ergo, a battery is made. To store the energy in the battery for long term use, the energy of the electrons is used to make chemical bonds between carbon, oxygen, hydrogen and often, nitrogen.
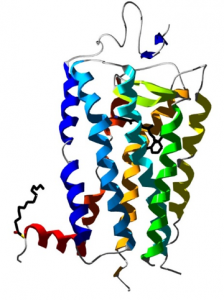
Figure 1: Structure of rhodopsin. The long helical structures represent 7 protein segments that span a membrane making a barrel-shaped structure. Membranes are made of oil, and the only way for water-soluble ions to get across is through the central pore in the barrel controlled by retinal (seen as a hexagonal ring to the left of the green strand). When the 11-cis form is present, the pore is open. When the all-trans form is present, the pore closes. Image: S. Jähnichen, Public Domain. https://commons.wikimedia.org/wiki/File:Rhodopsin.jpg For an interactive experience that will instantly turn you into a protein structure nerd, visit http://www.rcsb.org/3d-view/1E0P.
Going back to light: harvesting light energy requires resonance transfer – the flipping of molecules between one form and another. In an earlier article, I introduced retinal as a common resonance molecule in all opsins: bacteriorhodopsin, rhodopsin, photopsins, melanopsins, and the recently discovered heliorhodopsin [1]. Chlorophyll also harvests light by resonance transfer, but there are some small differences in structure and function. Chlorophyll has an undesirable side effect – it releases oxygen from water, which is a the plant equivalent of trash. Our anthropocentric tunnel vision makes us think oxygen is a benevolent molecule. It is not; it is the reason why antioxidants are a huge nutraceutical business. But photosynthetic organisms have hacked eukaryotic metabolisms into recycling oxygen trash to valuable carbon dioxide, the stuff of which sugar-batteries are made. Therefore, in bacteria, fungi and plants, which do not have eyes, retinal helps to channel the power of light to make sugars, starch and oils, and to thus store the sun’s energy for a rainy day. In profligate animals however, this power is instead used to send messages to a light-sensitive screen to help see well enough to take food away by force from bacteria, fungi, plants, or even other animals. And there you have the biggest surprise of them all: Animals have the same fundamental light-sensing mechanism in their eyes that bacteria and plants use in their photosynthetic pathways. If you don’t think that is a cooler storyline than the plot of the latest Star Wars movie, I give up.
As to the light-sensitive screens themselves, they work like the screens of our computers, phones and movie theaters. They may be of different sizes and shapes, but they all essentially do the same thing – produce pixelated images based on light and dark and color based on the type of screen and the light-sensitive opsin molecules that are present. We call these screens “retinas” and we are dependent on them for our predatory lifestyle. A simple animal that has eyes is the flatworm, an insignificant creature to us, but actually a predator of astonishing ability. [The internet hosts a whole series of horror movies starring predatory flatworms. Here’s one: https://www.youtube.com/watch?v=3DU_pvAtIYQ]. The head has a small region where a multitude of photoreceptor (light-sensing) cells containing retinal-containing opsins accumulate and which carry signals to a very primitive brain. Flatworm photoreceptors can only distinguish light and dark, but even that’s better than being completely blind. Over time, the areas of photoreceptor cells expanded, became concave, enclosed fluid and eventually developed a lens that focuses light. The specific steps of eye evolution are well established [2] and beyond the scope of this article, but Figure 2 below shows a schematic of the progressive steps of the process. Eyes are not that hard to make. It’s like taking a bit of dough, stretching it, bending it, folding it and adding raisins to it. Elementary stuff for nature’s baker.

Figure 2: Evolution of the simple eye. Attribution: Matticus78 at the English language Wikipedia. Modified from the original. This file is licensed under the Creative Commons Attribution-Share Alike 3.0 Unported license.
So what about variations in types of eyes? There are two basic types of eyes: simple eyes, and compound eyes, with different levels of complexity within each. The other variable is lens flexibility. If the lens is not flexible, then you have to change the focal distance of lens from the retina, as if you were using a telescope. Simple eyes have one lens and one retina and produce one image. Fortunately, our simple vertebrate eyes have high visual acuity due to the ability of the lens to become thicker or thinner on demand, except for those of us who wear glasses due to myopia or hypermetropia, because our lens are not as flexible as of those who have 20/20 vision.
Compound eyes are really like a bunch of smaller eyes stuck (compounded) together to produce either multiple images or a single, integrated image. Compound eyes that produce independent images that are later integrated in the brain are found in Strepsipteran insects which have terrific visual acuity. [I will talk about these astonishing and bizarre animals in a future article – prepare for a creepier version of Invasion of the Bodysnatchers.] The compound eyes of insects such as bees, called ommatidia, fold over the side of the bees head, extending from the top all the way down the side. What I love most about these compound eyes is that if you look closely, each smaller lens takes up a hexagonal shape, the same shape as the cells in a comb. The explanation is simple: it’s that a hexagonal shape is the most efficient packing shape, but it’s hard not to get carried away by the unexpected harmonizing. Bees additionally have three smaller single eyes called ocelli. For a fun and interactive way to see like a bee, visit B-EYE by Andy Giger at http://andygiger.com/science/beye/beyehome.html.
Two other short observations to finish off this article: 1. The best eyesight in terms of color spectrum and acuity belongs to the humble mantis shrimp. 2. Eyes have been adapted to sensing the infra-red wavelength, also known as heat, by pit vipers and some types of shrimp.
Just imagine that: pit vipers and shrimp used night-vision for hunting way before humans ever thought of it.
References:
- Béjà, O. Deep purple functional metagenomics. 2018; Available from: https://naturemicrobiologycommunity.nature.com/users/56173-oded-beja/posts/33088-deep-purple-functional-metagenomics.
- Arendt, D., H. Hausen, and G. Purschke, The ‘division of labour’model of eye evolution. Philosophical Transactions of the Royal Society B: Biological Sciences, 2009. 364(1531): p. 2809-2817.